Organic and Carbon Aerogels
What is an Organic Aerogel?
Organic aerogels have been around as long as any aerogel-in fact, the first aerogel Samuel Kistler is believed to have prepared was aerogel made from jelly (which is composed of the organic heteropolysaccharide pectin). Kistler also prepared aerogels of gelatin and rubbers, both of which are composed of organic polymers.
Basically, an organic aerogel is any aerogel with a framework primarily comprised of organic polymers. Generally, organic aerogels have very different properties from inorganic aerogels such as silica aerogel and metal oxide aerogels. They are generally less friable and less fragile than inorganic aerogels, instead squishing when compressed. The term “organic aerogel” can refer to one of many different kinds of aerogels, each with properties arising from the polymer which makes up the aerogel’s framework.
Organic aerogels can be made from resorcinol formaldehyde, phenol formaldehyde, melamine formaldehyde, cresol formaldehyde, phenol furfuryl alcohol, polyacrylamides, polyacrylonitriles, polyacrylates, polycyanurates, polyfurfural alcohol, polyimides, polystyrenes, polyurethanes, polyvinyl alcohol dialdehyde, epoxies, agar agar, agarose, and many others (see C. S. Ashley, C. J. Brinker and D. M. Smith, Journal of Non-Crystalline Solids, Volume 285, 2001).
Although organic aerogels have been around since the first aerogels were prepared, they were, for the most part, overlooked until the 1980’s when Lawrence Livermore National Laboratory scientists began producing organic aerogels made of phenolic resins. The bulk of this work was done by scientists Dr. Rick Pekala and Dr. Joe Satcher, who synthesized the first resorcinol-formaldehyde polymer aerogels (or RF aerogels for short).-essentially, aerogels composed of the same material as the plastic “Bakelite”. Depending on their density, RF aerogels range from light orange to deep red to black in color and range from translucent to opaque. Low density organic aerogels (<0.020 g cm-3) are generally irreversibly squishy, similar in feel to green floral potting foam. High density organic aerogels (>0.5 g cm-3) can be extremely robust and very hard to squeeze, almost like a car seat cushion.
What is Carbon Aerogel?
Not long after RF aerogels were developed, Livermore scientists discovered that by heating them to temperatures of several hundred degrees Celsius in an inert atmosphere (such as nitrogen or argon), the polymer which makes up the aerogel can be dehydrated (or “pyrolyzed”) to leave behind an aerogel made of carbon! As the name might suggest, carbon aerogels are totally black and not transparent. To the touch they are very similar in consistency to activated charcoal like the type you’d use for an aquarium filter. Today, organic aerogels made from polymers of not only resorcinol but also melamine, phlorglucinol, and acetic acid are used to prepare carbon aerogels.
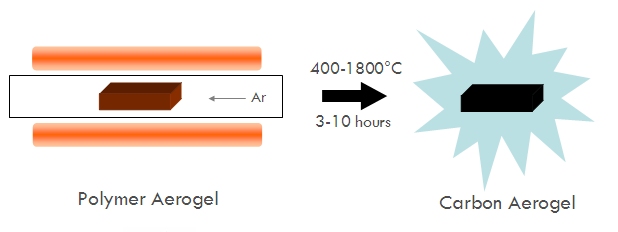
Pyrolysis of Many Organic Aerogels, Such as Resorcinol-Formaldehyde Polymer Aerogels, Yields a Carbon Aerogel
At the nanoscale, carbon aerogels are composed of nanoparticles of carbon with diameters approximately 1-2 nm. Like other aerogels, carbon aerogels are primarily mesoporous with a mean pore diameter of approximately 7-10 nm typical. Most carbon aerogels have a surface area ranging from 500-800 m2 g-1 however this is highly dependent on density and whether or not other stuff has been introduced (intentionally or unintentionally) into the aerogel. The surface area of a carbon aerogel can be easily increased post-production by placing it under a flow of steam or hydrogen at elevated temperatures (400°C-1000°C). At these temperatures, water and hydrogen will react with carbon in the aerogel to form gaseous products and eat micropores (pores <2 nm in diameter) throughout the interior of the aerogel, thereby increasing their surface area up to 2 500 m2 g-1.
Carbon aerogels are exciting materials because they not only have surface areas ranging from about 500 to 2 500 m2 g-1 but they can also be electrically conductive! The combination of these two properties makes carbon aerogels valuable for applications which benefit from high-surface-area electrodes, such as supercapacitors, fuel cells, and desalination systems.
Synthesis of Resorcinol-Formaldehyde Aerogels
By far, RF aerogels are the most researched organic aerogels and so we’ll use them as an example of how organic aerogels are prepared. Like other kinds of aerogels, RF aerogels are prepared through a sol-gel process, starting with a solution containing small molecules with the ability to link together (polymerize) to form larger molecular clusters that can eventually grow into nanoparticles dispersed throughout the solution (a sol). These nanoparticles can then be coaxed into interconnecting (or as they say in polymer chemistry, cross-linking) to form a continuous network of interconnected nanoparticles that spans the volume of the liquid solution, namely, a gel.
In the case of RF aerogels, the chemistry that is used is the polymerization of resorcinol (properly called 1,3-dihydroxybezne) with formaldehyde (properly called methanal) in water. This reaction is impractically slow at room temperature and so a small amount of a basic catalyst such as potassium or sodium carbonate is usually added. Over the course of about 24 hours at room temperature, polymer chains of resorcinol-formaldehyde polymer will form throughout the solution, tangling up into RF nanoparticles. The solution is then put into an oven (in a sealed bottle or mold!) at 80°C for 1-3 days (or just left at room temperature for a couple weeks), during which the nanoparticles cross-link together to form a mesoporous network across the solution, resulting in a gel.
The resulting RF gel has a consistency much rubberier than silica aerogels, more like canned cranberry sauce, and can even be sliced as such. Just like other aerogels, the RF gel must be purified before supercritical drying. For RF gels, this entails a few soakings in water, followed by a few soakings in a polar organic such as acetone or ethanol to prepare for supercritical drying. Lastly, the gel is supercritically dried to produce RF aerogel.
Other Organic Aerogels
There are numerous examples of organic aerogels which have been prepared in the literature, many of which are biological in nature. Supercritical drying has been used by biologists for many decades to preserve biological specimens or prepare them for electron microscopy-in essence making aeroinsects, aerobacteria, even aeroorgans! Biological materials are often nanostructured and frequently contain water-filled nanoporosity which causes the material to shrink upon dehydration. But by replacing the water in the pores of the material with, say, ethanol, and then replacing then ethanol with liquid CO2, the specimen can be supercritically dried at biologically-compatible temperatures leaving behind a dry but otherwise in-tact sample. This technique has even been used with collagen-based gels to prepare grafts for artificial skin.
The range of organic gels possible is vast so keep in mind-if you can make it into a gel you can probably make it into an aerogel, and aerogels often can do things wet gels can’t.
Derivation of Carbon Aerogels through Pyrolysis of Organic Aerogels
Carbon aerogels are relatively easy to make once you have a suitable organic aerogel. Basically, the aerogel is placed in a box furnace or into a quartz tube placed inside an electric clamshell furnace. A controlled flow of an inert gas on the order of 200 sccm of nitrogen or argon is flowed through the furnace or quartz tube throughout the whole process. The furnace is then heated to a target temperature, ranging anywhere from 400°C to 1100°C but sometimes as high as 1400°C to 1800°C. The sample is left in the furnace at elevated temperature for anywhere from 1-12 hours, during which the organic polymer expels much of its non-carbon content (such as oxygen, hydrogen, and nitrogen) leaving behind a carbonized skeleton in the same shape as the original organic aerogel. Usually an aerogel monolith will shrink during pyrolysis, but because it also loses mass from the expulsion of non-carbon contents, the density of the carbon aerogel often ends up being about the same as the density of the original organic aerogel.
Applications of Carbon Aerogels
One of the major interests in carbon aerogels is making electrical conductors with high surface areas. One example of why this is useful is for production of supercapacitors.
A capacitor is an energy storage device that stores energy as a static electric field which can rapidly release its stored energy over a relatively short period of time (fractions of a second). Capacitors are used in camera flashes to enable discharging enough charge to produce a quick but bright flash of light to illuminate the scene of interest (if you’ve ever heard the high-pitch sound the camera makes during a flash charge, that’s the capacitor). Capacitance is measured in units of farads (F). A one-farad capacitor is a pretty big capacitor and is capable of running a flashlight bulb for about five minutes (and giving you a nasty shock!). At its most basic, a capacitor is composed of two parallel conductive plates separated by a gap composed of air or an insulating material such as Nylon® or paper.
The equation that governs how much energy a capacitor can store is approximately
C = εA/d
where C is the capacitance, ε is the dielectric constant of the separator between the plates, A is the surface area of the plates, and d is the distance between the plates. The larger A is in this equation, the more energy the capacitor can store-and for applications where infinitely-large plates are too cumbersome to carry around, high-surface-area conductors like carbon aerogels are very important materials.
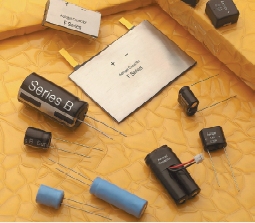
PowerStor(R) Supercapacitors Contain Carbon Aerogel for Their Ultrahigh Surface Area Electrodes (image courtesy Cooper-Bussman)
Since carbon aerogels can have such incredibly high surface areas and electrical conductivity, they are now used as the electrode material in supercapacitors, namely the PowerStor® line produced by Cooper-Bussmann. In fact, in the early 1990’s, the largest component capacitors that you could buy that would fit in the palm of your hand were about 500 millifarad to 1 farad in capacitance. By the end of the 1990’s, thanks to the commercialization of carbon aerogels, capacitors of 50 to 100 farads that could fit in your hand had become available-this is kind of like the buying a 300-horsepower BMW and then suddenly a 15,000-horespower engine comes out! Capacitors with such high capacitance are called supercapacitors (or sometimes ultracapacitors).
Because supercapacitors can now carry so much energy, people are now experimenting with the idea of using supercapacitors as replacements for batteries in some devices and systems. Although supercapacitors do not yet have the same energy density as commercial batteries, they can charge and discharge much more quickly than batteries, making them useful for things like hybrid vehicles and storing energy from variable power generating devices like solar panels and wind mills. People have even proposed next-gen supercapacitors for powering cell phones-imagine if it only took a few seconds to charge your phone but in exchange you had to charge it two or three times a day-an interesting alternative to a battery.
Carbon Aerogels and Their Conspiracy to Thwart Conductivity
Like graphite and charcoal, carbon aerogels are sort of but not very electrically conductive-certainly not as good as most metals but definitely much better than insulators like plastics or glass. Carbon aerogels, however, have an interesting feature in that their electrical conductivity is a function of their density.
It turns out to increase the surface area of a carbon aerogel, you have to reduce its bulk density. Low-density carbon aerogels (<0.02 g cm-3) have a different morphology (nanostructure) than high-density carbon aerogels in that they have thinner struts, a different distribution of particle sizes, and less internal connectivity. This, in turn, changes the way the material can transport electric charge. As a result, the highest surface area carbon aerogels end up having the worst electrical conductivity, and the most conductive carbon aerogels have lowest surface areas. For using carbon aerogels in applications where we want both high surface area and good electrical conductivity, this means figuring out how to maximize electrical conductivity and surface area simultaneously.
Scientists have developed a number of ways to combat this conspiracy of conductivity. One way is by activating high-density carbon aerogels, namely by exposing them to steam or hydrogen at temperatures of 400-1,000°C (as described above). At these temperatures, steam and hydrogen eat away at the aerogel’s structure in effect revealing ultrasmall micropores (pores <2 nm in diameter) thereby giving the aerogel higher surface area. By starting with a dense aerogel and etching it in this way, a better electrical conductivity and higher surface area is attained than if a low density aerogel was prepared directly through dilution of the gel precursor solution. Another method to improve electrical conductivity in carbon aerogels is to introduce conductive materials into the aerogels, such as doping them with metals.
Metal-Doped Carbon Aerogels, or, Nano Blueberry Muffins
In the early 2000’s, Dr. Ted Baumann at Lawrence Livermore National Laboratory developed a technique for growing nanoparticles of metals inside carbon aerogels through a very clever technique. In this technique, resorcinol is replaced with 2,4-dihydroxybenzoic acid (DHBA), which is basically resorcinol with a carboxylic acid group attached to it. The DHBA (a weak acid) is then neutralized with potassium carbonate (a strong base) in water to produce potassium 2,4-dihydroxybenzoate (a water-soluble salt). Formaldehyde is added and the solution is cured at 80°C in sealed molds for 1-3 days. The resulting cranberry-sauce-red gels are similar to RF gels used to prepare carbon aerogels, except for that they are laced with potassium ion exchange sites throughout their structure. Soaking these gels in a solution of a metal salt, such as iron(III) nitrate, results in exchange of potassium ions with incoming metal ions which then attach to the gel’s polymer backbone. Think of it like hanging ornaments on a Christmas tree, where the ornaments are metal ions and the Christmas tree is the gel’s framework. The metal-doped gels can then be supercritically dried to make metal-doped organic aerogels and, finally, pyrolyzed to produce carbon aerogels. However, unlike typical carbon aerogels, during pyrolysis the added metal ions attached to the aerogel’s backbone reduce to metal atoms and coagulate into nanosized particles. The resulting material can be thought of kind of like a blueberry muffin morphology in which metal-containing nanoparticles (blueberries) are dispersed throughout a nanoporous carbon matrix (muffin). The presence of these particles does a number of things, for example, improves the electrical conductivity of the aerogel (compared with an undoped carbon aerogel of the same density), and gives the aerogel some catalytic properties characteristic of the dopant metal.
Since the chemistry for doping the gels is done in water, a number of metals can be used to prepare metal-doped carbon aerogels including iron, cobalt, nickel, and copper.
In 2005, Aerogel.org’s own Stephen Steiner at MIT and Dr. Ted Baumann at Lawrence Livermore discovered that by using a chemical vapor deposition technique, it was possible to grow carbon nanotubes from the nanoparticles in Fe-doped carbon aerogels, resulting in nanotube growth on the outer surfaces of the aerogels (but not inside the pores). Later the metal-doping technique was extended to virtually any metal on the periodic table and it was found that using metal-doped carbon aerogels it was possible to grow nanotubes from a number of metal catalysts previously not known to catalyze nanotube growth.
The Future of Carbon Aerogels – Energy Storage Applications
Many exciting developments in the area of energy storage involve carbon aerogels. Dr. Jeff Long and Dr. Debra Rolison at the Naval Research Laboratory have prepared 3-D hybrid electrochemical storage devices with carbon aerogel materials-something in between a battery and a supercapacitor that can scale in three dimensions instead of just two! Their materials involve a high-surface area carbon substrate with a conformal coating of a dielectric upon which is a conformal conductor coating-effectively intertwining two conductive aerogels in the same volume! Dr. Ted Baumann at Lawrence Livermore has been doing some very interesting work on hydrogen storage on carbon aerogels, and has found high-surface-area carbon aerogels to be an effective storage medium for hydrogen (although not quite at room temperature yet).
Lots more developments to come from carbon aerogels in the near future. Stay linked!